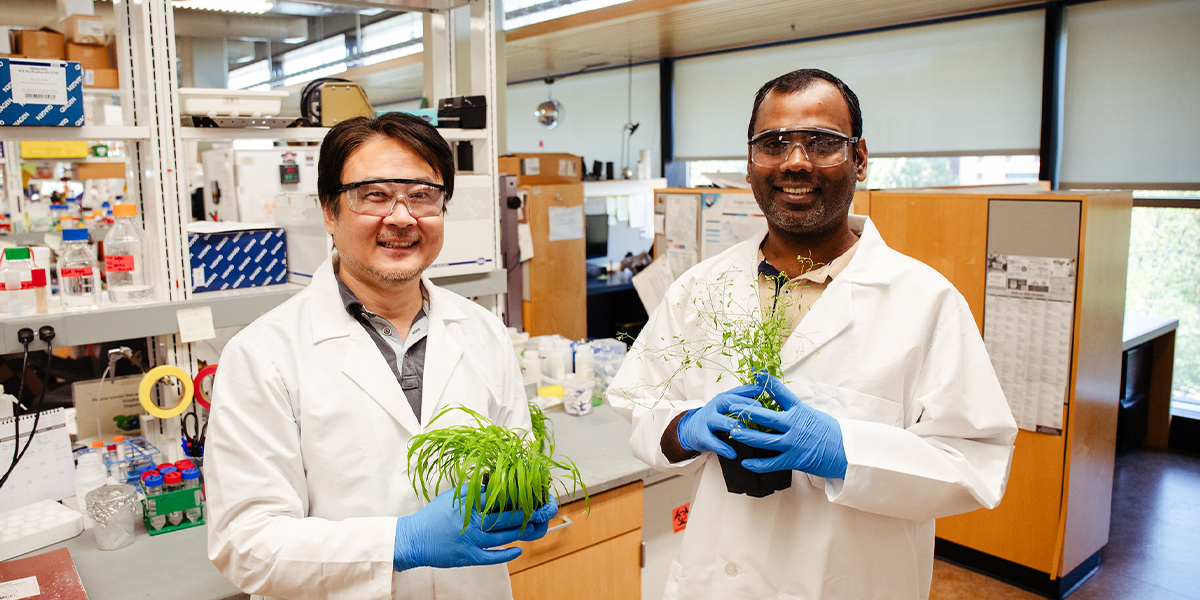
Feng Zhang (left) and Jitesh Kumar (right) work with their two model species in the Cargill building.
Traditional plant breeding is slow and methodical. Imagine a scientist in the field, initiating a breeding program by transferring pollen from one flower to another with a paintbrush. Developing a variety this way can take years. Over the past decade, however, CRISPR gene editing has emerged as an option for fast-tracking new crop development in a time of cascading climate-induced agricultural threats. Now, a recent breakthrough makes this gene-editing technique even more effective.
“The bottleneck for gene editing in plants was, yes, we can disrupt the genes, but we could not be very precise in controlling the outcomes,” says Feng Zhang, an associate professor in the Department of Plant and Microbial Biology. “A lot of CRISPR tools are based on human data, so when we try to extend that to plant research, it didn’t work well.”
Zhang delved into the discrepancies between plant and human gene-editing results with graduate students Trevor Weiss and Jitesh Kumar. Their findings with other lab members and colleagues, recently published in Nature Communications, reveal that the accuracy of gene editing in plants can be fine-tuned by manipulating a repair enzyme called DNA polymerase lambda.
The discovery emerged from a series of experiments involving two plant species: Arabidopsis, a well-studied species related to commercial crops like mustard and canola, and Setaria, a grass that has similar characteristics to corn and millet. “We cannot assume gene-editing techniques that work in one type of plant will always work the same way in another,” Zhang says, explaining the rationale behind testing both plant species. Indeed, while the species responded much more similarly to one another than to human cells, the gene-editing results in both plants sometimes appeared to be random.
The team explored how a variety of factors affected these outcomes, including the way DNA is bundled with proteins inside the cell. The most significant breakthrough came when they identified the role of DNA polymerase lambda. When they removed this repair enzyme, the plant cells could not add new genetic material at editing sites, while overexpressing the enzyme greatly increased the rate of accurate genetic additions. In fact, by calibrating the amount of DNA polymerase lambda present in the cell, the team could precisely edit individual base pairs, which are the smallest units of DNA that can be altered.
Of course, gene editing crops may cause trepidation in some corners. Previous genetic engineering methods involved splicing genes from one species to another, driving public concern about whether the resulting GMO foods were safe to eat. In comparison, CRISPR resembles traditional plant breeding in that it tweaks the existing genetic sequence without introducing genes from other species, and the DNA polymerase lambda discovery makes this nipping and tucking process even more surgical. “If we talk about genetically modified organisms, our corn has been genetically modified for 10,000 years,” Zhang points out. “The DNA has been changed compared to their wild relatives.”
Zhang is now partnering with the Cereal Disease Lab, a US Department of Agriculture station on the University of Minnesota campus, to apply new gene-editing technologies for small grain crops, such as wheat, barley, and oats. “The goal is to quickly generate more climate-resilient or disease-resistant crops,” Zhang explains. In an era of record-setting temperatures, extreme climate patterns, and globetrotting pests and diseases, such agricultural advancement couldn’t come quickly enough. –Jonathan Damery